Space vehicles
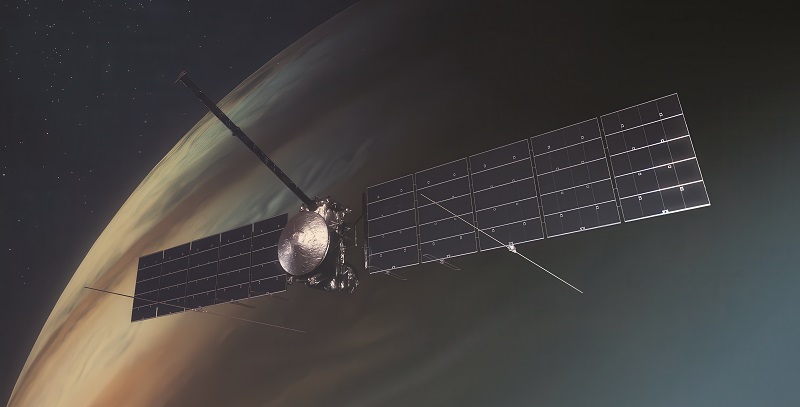
Nick Flaherty investigates the revolutionary projects taking uncrewed crafts into deeper space
The next few years will see a dramatic move forward for autonomous space systems. Several key projects are launching, sending new types of spacecraft into orbit and far out into the solar system to investigate Europa, a moon of Jupiter.
One of these is Dream Chaser, a smaller version of NASA’s Space Shuttle; a winged autonomous spacecraft that can recover fragile payloads in orbit.
The first version of Dream Chaser is called Tenacity and represents an uncrewed spiritual successor to the shuttle. At 9 m long, it is roughly a quarter of the total length of the Space Shuttle, with a pressurised volume of 33 m3 (including the spaceplane and cargo module). The design supports gentle runway landings with an acceleration of 1.5 g to recover fragile cargo, rather than the land or sea landings by parachute of other reusable modules.
The launch of Tenacity is now planned for 2025 using the United Launch Alliance (ULA) Vulcan Centaur rocket. The craft is designed to fit into the 5 m-wide fairing on the top of the launcher and the wings unfold, and it is intended to be reused at least 15 times.
The final assembly and system checks include the installation of the remaining thermal-protection system tiles, as well as propulsion system closeout and leak testing. Electromagnetic interference (EMI) and electromagnetic compatibility (EMC) testing ensures all of the electrical components function in space’s challenging radio-frequency (RF) environment.
Additionally, acoustic testing will verify that both Dream Chaser and its cargo module can withstand the intense noise levels experienced during launch.
Runway testing will verify Dream Chaser’s differential braking system upon returning from space and landing at Space Florida’s storied Launch and Landing Facility onsite at Kennedy.
Sierra Space uses AI for spacecraft design and complex data-management systems for real-time data analysis. This adaptive software processes substantial volumes of information for predictive maintenance and comprehensive health management, so mission control can monitor systems in real time, vastly improving situational awareness and spacecraft safety.
The technology’s adaptability extends to real-time tracking of space debris, enhancing collision avoidance measures, and supporting autonomous navigation and communication systems.
This isn’t the only autonomous winged craft in orbit. The US military has the X37-B, a similar design that is also a quarter of the size of the Space Shuttle.
The avionics are designed to automate all de-orbit and landing functions. The craft was built by Boeing with a lighter composite structure, rather than the traditional aluminium, and a new generation of high-temperature wing leading-edge tiles and toughened uni-piece fibrous refractory oxidation-resistant ceramic tiles. The flight controls and brakes use all electro-mechanical actuation with no hydraulics.
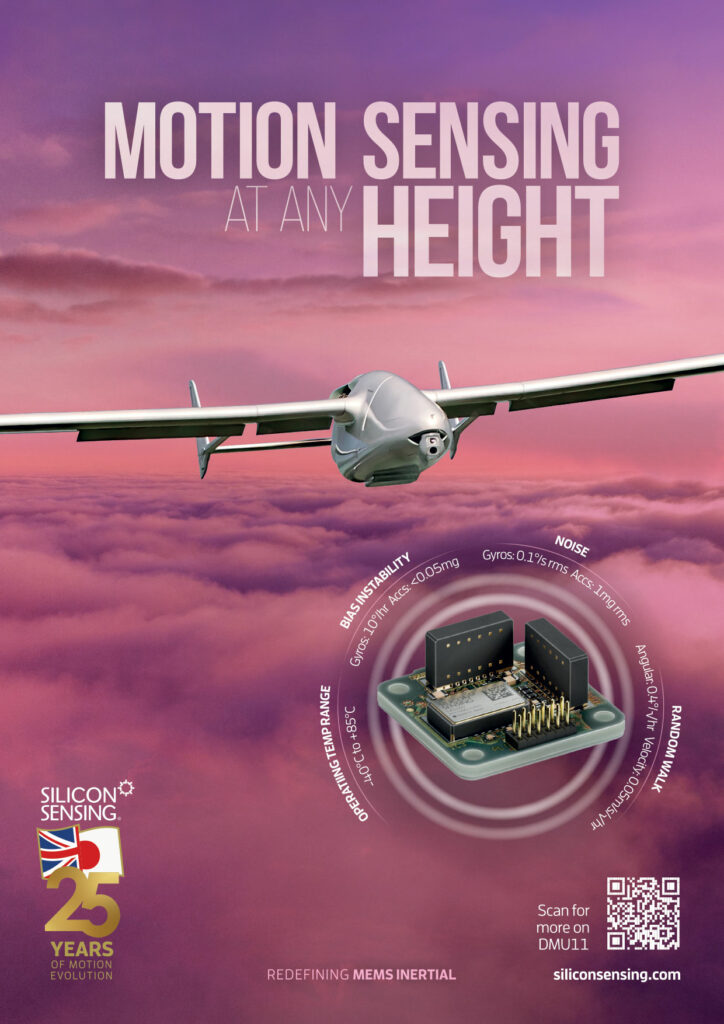
The craft is designed to stay in orbit for 207 days, but it operated for over 900 days during its sixth mission in 2022, carrying experiments for the US military.
Meanwhile, UAV maker Anduril Technologies is looking to use its avionics technology for space systems. The company aims to develop fully integrated hardware and software systems based on its Lattice software platform to autonomously monitor and manage space-based assets, using AI with modular mission payloads.
Anduril aims to develop its own fully integrated systems by the end of 2025. This mission will serve as a testbed for maturation of multiple Anduril and third-party payloads.
For systems in orbit, Aethero has teamed up with Antmicro to use open-source tools to develop hardware for AI in space applications. They are developing flight-ready modular edge computers that can handle space AI and data processing on satellites. The software and hardware co-development workflows use open-source plus an automation-driven approach to all parts of the stack to reduce design and development times.
Aethero has developed the space-qualified NxN Edge Computing Module as a radiation-hardened computing platform, which has its roots in Antmicro’s open-source Nvidia Jetson Orin Baseboard. This was developed with an open hardware design flow with the Antmicro KiCad and Blender tools.
Antmicro provided the basis of both the Linux and Zephyr RTOS software controlling the payload, and implemented its own open-source RDFM framework for a modular, configurable platform.
The complete software stack for the onboard system was built and tested with Renode, Antmicro’s open-source simulator, to speed up the development process and increase testing coverage across a range of scenarios.
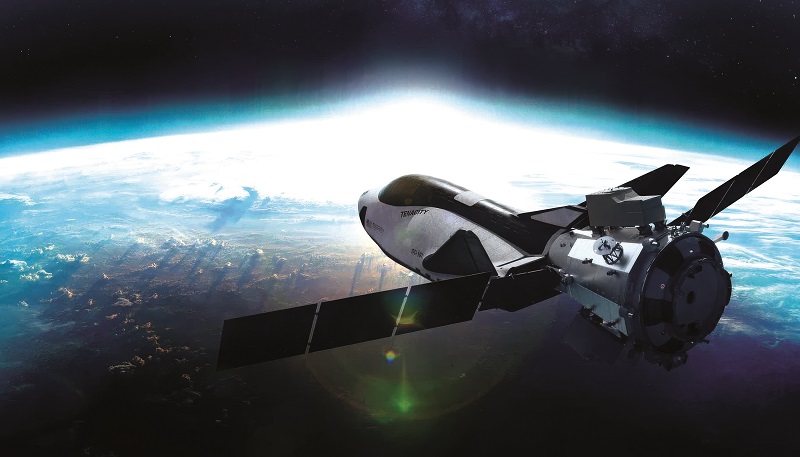
Swarms on Mars
A mission to Mars plans to use a swarm of robotic systems. Researchers at the University of Wuerzburg in Germany are developing a system that can explore an enormous canyon that stretches across the red planet. Valles Marineris is 3,000 km long, 600 km wide and an average of 8 km deep.
Since 2012, this largest known canyon in the solar system has been the focus of a project by the German Space Agency at the German Aerospace Centre (DLR).
The Valles Marineris Explorer (VaMEx) initiative aims to develop key technologies for robotic exploration of this difficult terrain in a complex swarm of driving, walking and flying autonomous vehicles. They will explore the canyon’s gorges and caves for the first time, and search for traces of liquid water.
“We have given our sub-project the name VaMEx3-MarsSymphony, because the aim is to make the individual elements of the robot swarm play together harmoniously, like an orchestra,” says project leader Professor Hakan Kayal.
In the current development phase, the swarm includes mobile robots in the air and on land, a stationary gateway on the ground that serves as a command centre for communications and a satellite simulator for data exchange with Earth.
When the robots on the ground enter caves they are shielded from the surface of Mars and cannot communicate directly with the gateway, so the concept includes repeater stations, which pass on the recorded images and data in a transport chain – from the robot in the cave to the gateway on the surface of the planet.
The swarm also includes autorotating UAVs that are dropped from the air and collect data as they glide gently to the ground. The elongated bodies are built like seeds with a single wing and rotate on their own axis, allowing them to gently spin downwards. Their flight path can be controlled so they can be distributed over a larger area, and then used as sensor, repeater and navigation networks.
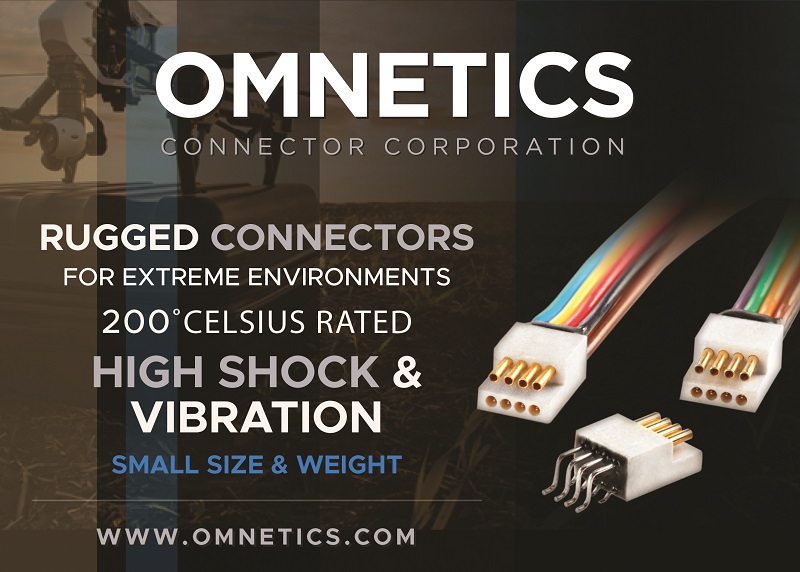
The stationary gateway will also be equipped with a camera that keeps an eye on the Martian sky.
“All previous Mars missions have focused on the surface of the planet, but we want to look upwards for the first time,” says Prof Kayal.
Meteorites the size of a basketball appear to hit Mars almost every day, as the researchers concluded in June from seismic data. “We could further substantiate this with data if we film the entry of meteorites with our camera and correlate these events with the seismic signals,” he says.
This Unidentified Anomalous Phenomena (UAP) camera uses AI to detect unknown celestial phenomena, such as those observed on Earth. The integration of a camera system for sky observation on the gateway represents a significant development step towards a detection system for short-term phenomena in the Martian atmosphere and for research into UAPs.
Communications between the elements described and the space segment is a key challenge in the transmission of the scientific data obtained. Due to scarce resources, this applies in particular to communications between the gateway on the surface of Mars and the relay satellites in orbit.
Current landers use the S-band or X-band frequencies. However, switching from the X-band to the Ka-band is a decisive step towards increasing the data rate of the transmission channel.
IQ Technologies for Earth and Space in Berlin, Germany, is developing a Ka-band capable transceiver for use on landers and small interplanetary satellites, based on its system called XLink, as well as customised and flexible transmission protocols.
The robot swarm will be tested next year, when participants will simulate the Mars mission on Earth, probably in a quarry in Germany. The Wuerzburg UAP camera will play an important role as the video recordings from the sky will provide sufficiently large volumes of data to test the resilience of the communications system.
Prof Kayal says that “in a possible follow-up project, the hardware would have to be adapted for use on Mars” to handle the thin atmosphere, -63 C average temperature and large dust storms that sweep across the red planet.
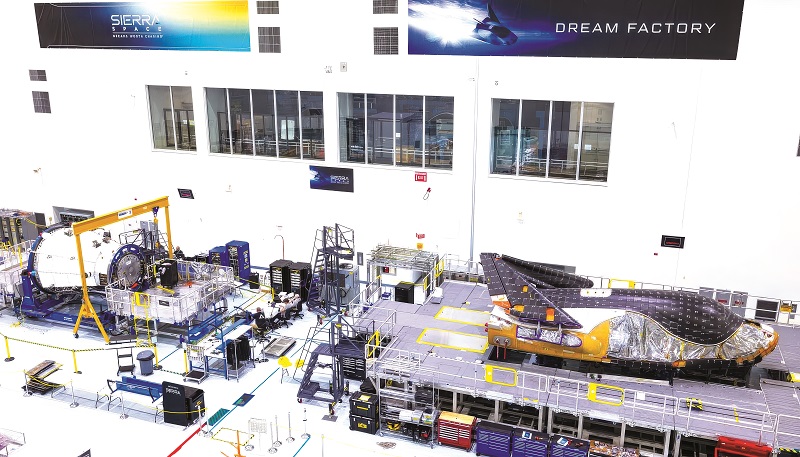
Deep space
Jupiter is about 480 million miles (about 770 million km) away from Earth. The Clipper mission to Jupiter’s moon, Europa, highlights the challenges of autonomous systems in deep space, particularly with radiation.
With its antennas and solar arrays fully deployed, Europa Clipper is the largest spacecraft that NASA has ever developed for a planetary mission. Bigger than a basketball court, it measures 30.5 m long and 17.6 m wide.
The main body consists of its avionics vault, RF module and propulsion module. At launch, it will weigh about 6,000 kg, with nearly half (2,750 kg) as fuel.
Mission planners will send Europa Clipper past Mars and then Earth using the planets’ gravity as a slingshot to speed the spacecraft’s trek, and after journeying about 1.8 billion miles (2.9 billion km) over five years, it will fire its engines to enter orbit around Jupiter in 2030.
Powering those flybys in a region of the solar system that receives only 3-4% of the sunlight that Earth gets is a challenge. The solar arrays need to be huge enough to collect sufficient light to power the craft’s instruments, electronics and other subsystems.
Each solar array is composed of five panels, and they were designed and built at Johns Hopkins Applied Physics Laboratory (APL) in Laurel, Maryland, and Airbus in Leiden, Netherlands.
At Jupiter, Europa Clipper’s arrays will together provide about 700 W, with batteries storing the power to run all of the electronics, a full payload of science instruments, communications equipment, the computer and an entire propulsion system with 24 engines.
While doing all of that, the arrays will need to operate in extreme cold conditions, as the hardware’s temperature will plunge to -240 C when in Jupiter’s shadow. To ensure the panels can operate in those extremes, engineers tested them in a specialised cryogenic chamber at Liège Space Centre in Belgium.
“The spacecraft is cozy. It has heaters and an active thermal loop, which keeps it in a much more normal temperature range,” says Taejoo Lee, solar array product delivery manager at APL. “But the solar arrays are exposed to the vacuum of space without any heaters. They’re completely passive, so whatever the environment is, those are the temperatures they get.”
About 90 minutes after launch, the arrays will unfurl from their folded position over the course of about 40 minutes. About two weeks later, six antennas affixed to the arrays will also deploy to their full size. The antennas belong to the radar instrument, which will search for water within and beneath Europa’s thick ice shell. These unfold to 17.6 m, perpendicular to the arrays.
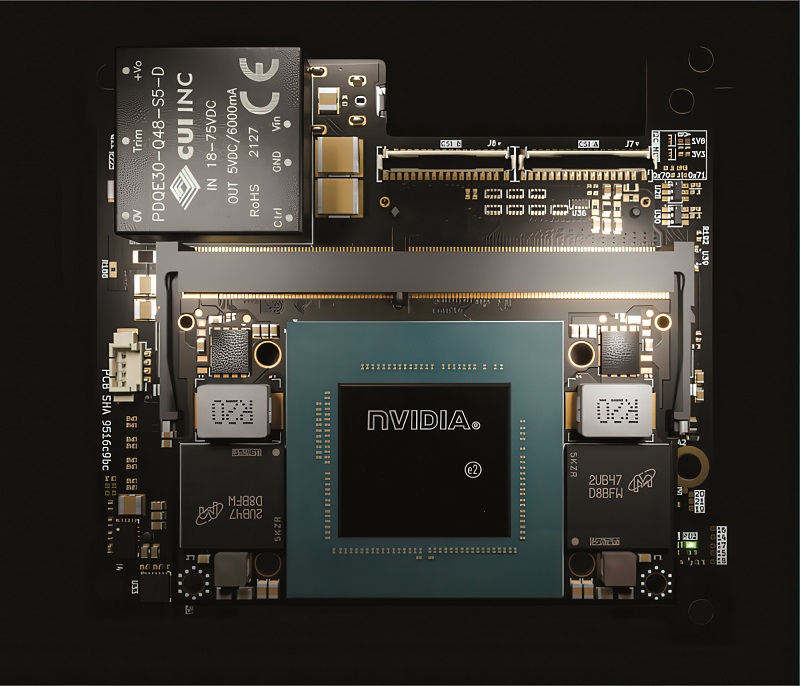
Radiation risk
Another key challenge for the power system is the radiation around Jupiter, the most intense in the solar system. The planet’s enormous magnetic field, 20,000 times stronger than Earth’s, traps charged particles and accelerates them to very high energies. When it circles the Europa moon, the craft will need to go in and out of these radiation bands.
NASA has been testing the radiation performance of power MOSFETs from Infineon Technologies. Those from Infineon’s acquisition of International Rectifier are used throughout the craft’s electrical power systems.
NASA engineers extensively tested the MOSFETs at the Jet Propulsion Laboratory after learning that some of these parts might not withstand the radiation around Jupiter.
Tests are also being conducted in Maryland, at APL in Laurel and NASA’s Goddard Space Flight Centre in Greenbelt. APL designed the main spacecraft body in collaboration with JPL and NASA Goddard.
The issue with the MOSFETs came to light in May, when the mission team was advised that similar parts were failing at lower radiation doses than expected. In June, Infineon issued an alert to notify users of this issue, and it worked with the mission team to support ongoing radiation testing and analysis efforts.
Test data showed some transistors are likely to fail as the parts are not as radiation-resistant as expected. However, the electronics are enclosed in a vault with walls made of 1/3 in-thick (9.2 mm) aluminium-zinc alloy sheets to protect them. The vault is bolted to the spacecraft’s propulsion module, which houses the 24 engines.
The MOSFETs are US DLA QPL products up to MIL-PRF-19500 JANS level and the hermetic packaging is screened to the European ESCC-5000 specification. The NASA team worked out how many MOSFETs may be susceptible and how they will perform in flight, and developed options for maximising the transistors’ longevity in the Jupiter system.
NASA says the issue that might be having an impact is a phenomenon the industry wasn’t aware of, which represents a newly identified gap in the industry standard radiation qualification of transistor wafer lots. However, launch preparations are progressing with the spacecraft at the Kennedy Space Centre in Florida, where the team recently attached the high-gain antenna.
When Clipper arrives at Jupiter in 2030, it will conduct science investigations to understand the potential habitability of the Europa moon as it flies by multiple times.
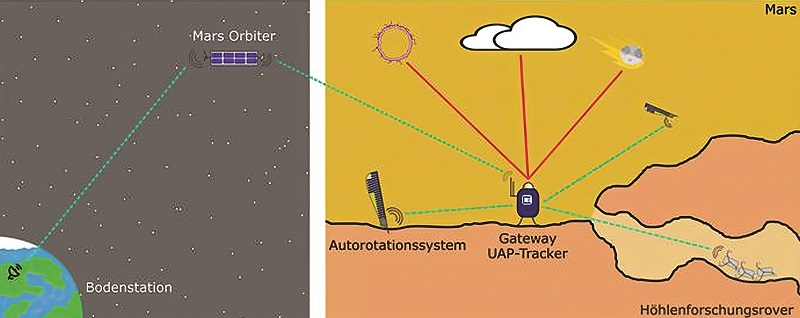
Precision flight
A European consortium is developing laser sensors to help satellites navigate with ultra-precision and enable uncrewed vehicles to fly further for longer.
The INPHOMIR project is developing two new ultra low-power, compact sensing devices – an optical gyroscope and a mid-infrared FMCW Lidar – with the aim of making space missions more efficient and cost-effective.
“As we aim to explore space much deeper while conducting more complex missions, the need for precise, reliable and efficient sensors is now more critical than ever. The advanced sensing technologies we are developing will hopefully enhance the accuracy of satellite positioning, improve navigation for interplanetary missions and ensure the success of space exploration,” says Daniele Palaferri, senior scientist at GEM Elettronica and project coordinator of INPHOMIR.
The team is developing an advanced optical gyroscope using laser diodes and indium phosphide sensors that integrate optical processing, These photonic integrated circuit (PIC) devices help to reduce size and power consumption, which are both key for space system designs.
The team is also developing a brand-new, mid-infrared, frequency modulated, continuous wave (FMCW) Lidar. This is similar to radar, only with laser to create detailed 3D maps of the environment.
“Think of a bat’s echolocation system, but for machines like drones and self-driving cars. Our FMCW is a fancy way of saying the Lidar sends out a continuous laser beam that changes its frequency over time. By doing this it can measure distances very accurately, even if objects are moving,” says Palaferri.
“In space missions, this technology can help satellites and rovers navigate and map out unknown terrains with precision. Unlike existing optical gyroscopes and mid-IR Lidar systems, INPHOMIR integrates all photonic components onto a single chip, reducing size, weight and power consumption. This means all parts of a sensor can be combined on a single chip and the overall device can be much smaller.
“By addressing big-data challenges through the development of data-fusion and AI algorithms, we aim to create solutions capable of handling massive flows of data.”
The multidisciplinary project is coordinated in Italy by GEM Elettronica and will conclude in 2027.
The scheme will validate the performance of the PIC devices with new, ultra low-loss waveguides and new, mid-infrared active devices. The first demonstrations of advanced building blocks, such as extremely high-quality factor resonators, narrow line-width quantum cascade lasers and ultra low-power, sensitive heterodyne detectors at mid-infrared wavelengths will follow.
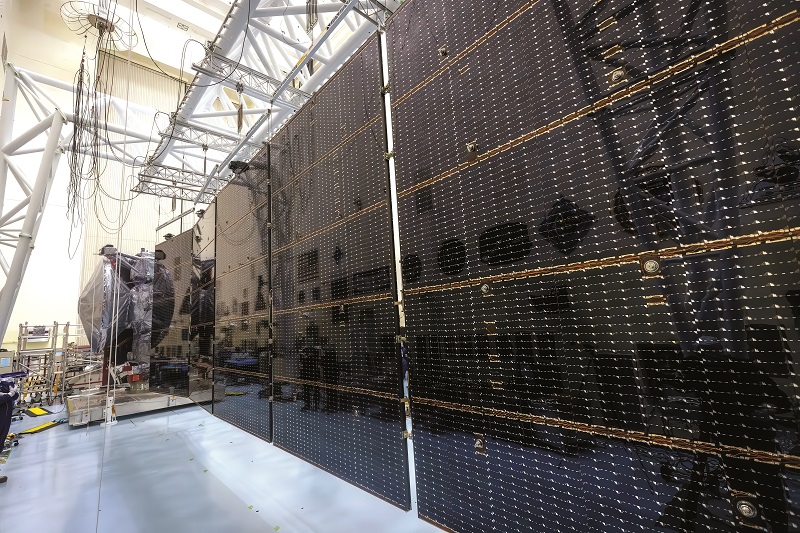
Atomic clocks
Navigating in deep space is not a trivial challenge. Using star maps is the natural way forward, but this requires precise timing over long periods of time, and that requires a lot of transmission data, which uses up the batteries.
Instead, a highly accurate onboard clock, called a one-way clock, frees up valuable bandwidth and opens up other applications in the deep space network that communicate with space craft. This would allow the network to simultaneously track two spacecraft on a downlink at destinations such as Mars, for example, and nearly double a space mission’s tracking data because it no longer needs to time-share an antenna. At Jupiter, this would yield a 10-15% increase in tracking, and at Saturn 15-25%, with the percentage rising the further a spacecraft travels.
Tracking data precision is improved by a factor of 10 by using the DSN’s Ka-band downlink capability and avoids weather sensitivity in the Ka-band.
NASA’s Deep Space Atomic Clock is a critical step towards enabling spacecraft to safely navigate independently in deep space, rather than relying on the time-consuming process of waiting to receive directions from Earth.
Launched in June 2019, the clock is being tested in orbit on a spacecraft provided by General Atomics Electromagnetic Systems of Englewood, Colorado. This is a key element of a real-time autonomous navigation system that tracks one-way radio signals on the uplink and, coupled with optical navigation, provides absolute and relative navigation data.
GPS data was collected through two years of testing in orbit, and this was used not only for precise clock estimation but also as a proxy for deep-space tracking data in an experiment on deep-space navigation.
Careful selection and processing of the GPS Doppler data and limited modelling fidelity representative of deep-space navigation capabilities showed the atomic clock could be used as a navigation instrument in conditions typical for a low-altitude Mars orbiter.
Onboard telemetry quantifying the ultrastable oscillator (USO) frequency correction is processed to show the orbit determination performance degradation when using one-way tracking data.
The atomic clock was 50 times more stable than those on GPS satellites, and it is also the first atomic clock that is small and stable enough to fly beyond Earth’s orbit. Timing and frequency stability is comparable to the deep space network’s ground clocks and it can make one-way measurements with similar accuracy to two-way tracking data.
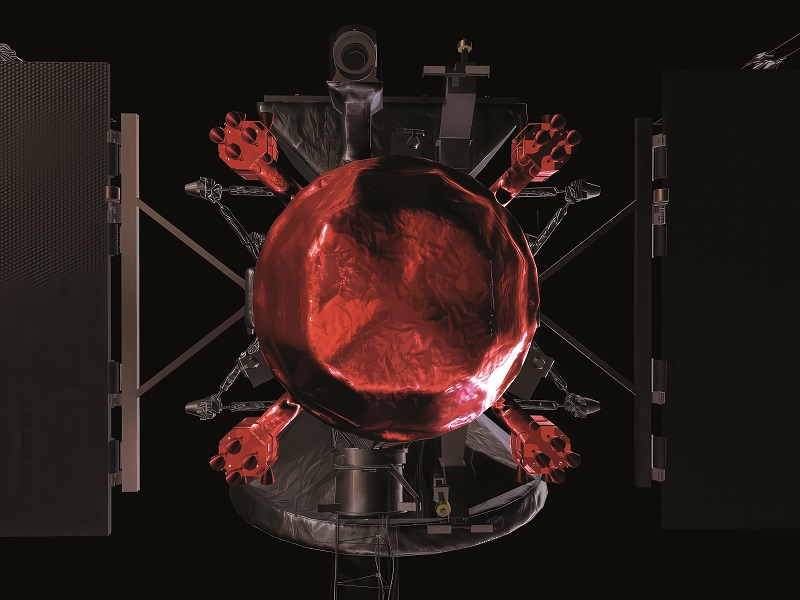
Going quantum
Another approach being developed is quantum clocks. These are the size of a rack unit (1U) at the moment, but scientists at the University of Birmingham have successfully tested a new technique for generating cold atoms that can be used for a smaller quantum clock.
The technique, developed by Aquark Technologies in Southampton, uses a laser cooling method called Supermolasses, which does not need an applied magnetic field, as required by other cold ion systems.
This lack of a magnetic field means a quantum clock can be made more portable and robust, and therefore easier to use commercially. The tests at the University of Birmingham are the first time that the Supermolasses method has been independently achieved and they were carried out as part of an Innovate UK-funded project called Gravity Array.
Another way to get accurate timing is to use the universe itself.
The DeepSpacePULSE project in Spain is testing the viability of a more efficient and autonomous navigation system using pulsars as clocks.
Astrophysicist Nanda Rea, based at Consejo Superior de Investigaciones Científicas (CSIC) in Spain, and her team locate compact pulsar stars as the basis of a navigation system. These can be used as perfect clocks with unprecedented stability, producing regular pulses of X-rays, effectively making them a GPS system in space.
A navigation system has already been developed and tested on the International Space Station by NASA. To enable the use of an X-ray pulsar navigation system in deep-space missions potentially lasting 100 years, the DeepSpacePulse team will work on a more precise, autonomous, light navigation unit, expected to be the size of a microwave oven and weigh about 10 kg.
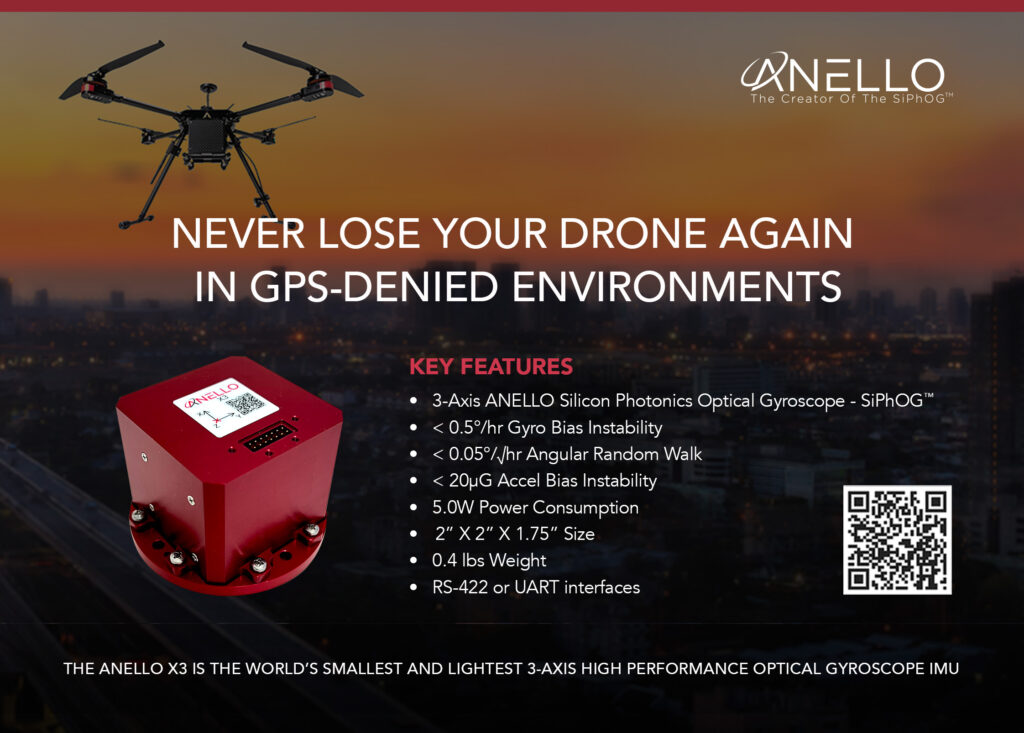
Looking forwards
Space systems take many years to launch, which makes the adoption of newer technologies such as AI and advanced navigation systems for autonomous operation slower. For example, the Dream Chaser has spent a decade in development, but in 2025 it will provide reusable, low acceleration, autonomous deliveries from orbit.
This is highlighted by the missions to planets further out in the solar system, such as Jupiter Clipper. Tackling the challenges of intense radiation for power systems has been a key issue with the programme, which, over the next five years, will reveal new insights into the icy moon of Europa.
The next generation of autonomous space systems will take advantage of photonic navigation systems, even using the X-rays from pulsar stars to provide the necessary timing data.
UPCOMING EVENTS
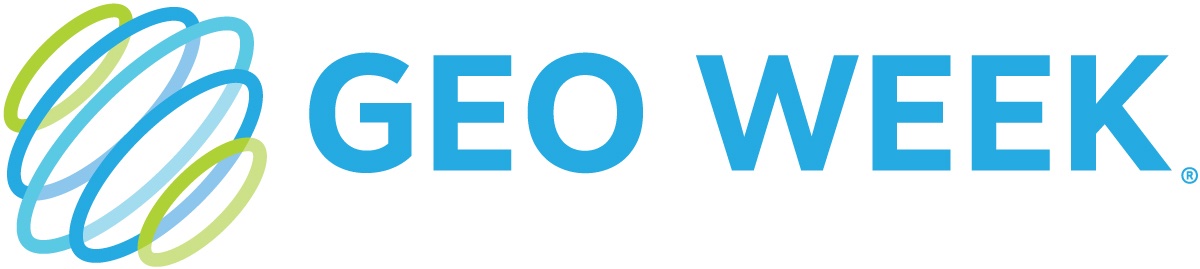
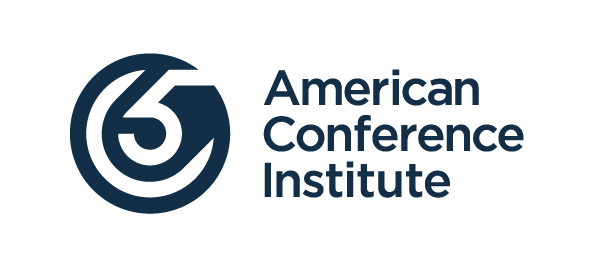
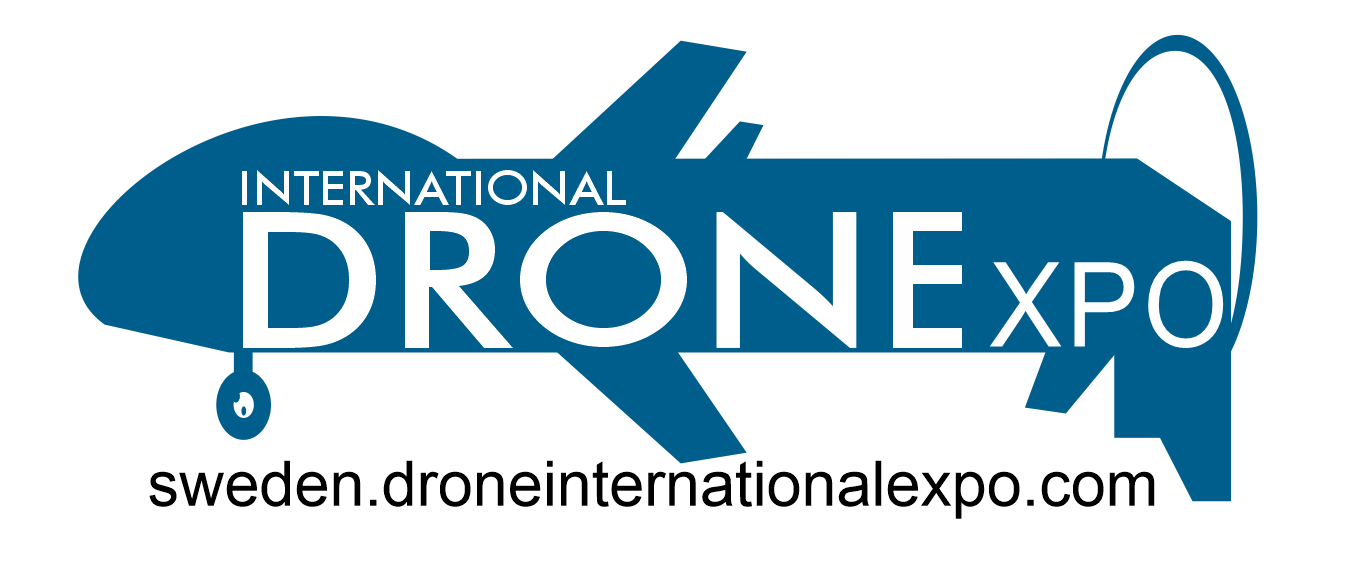
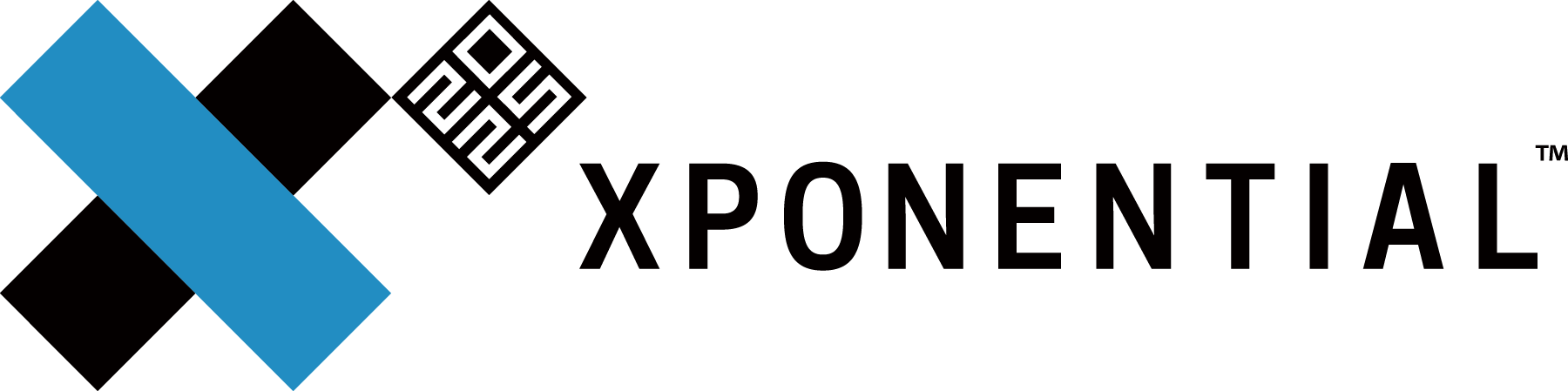
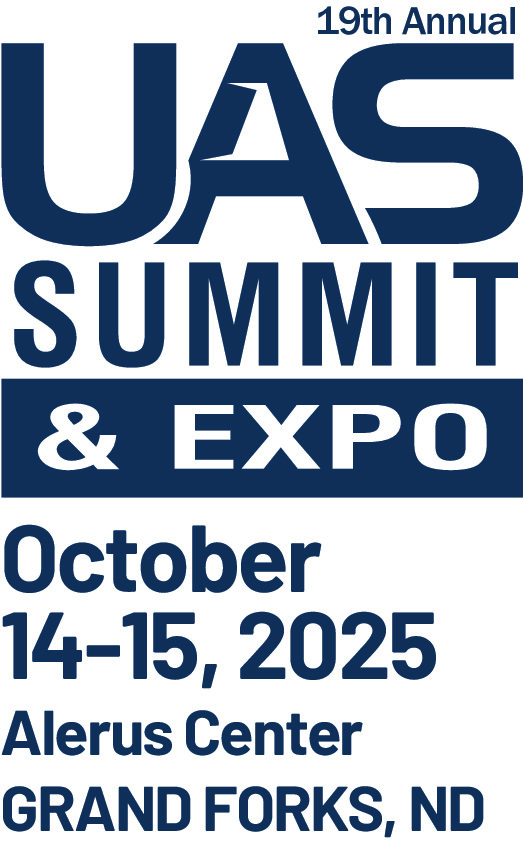

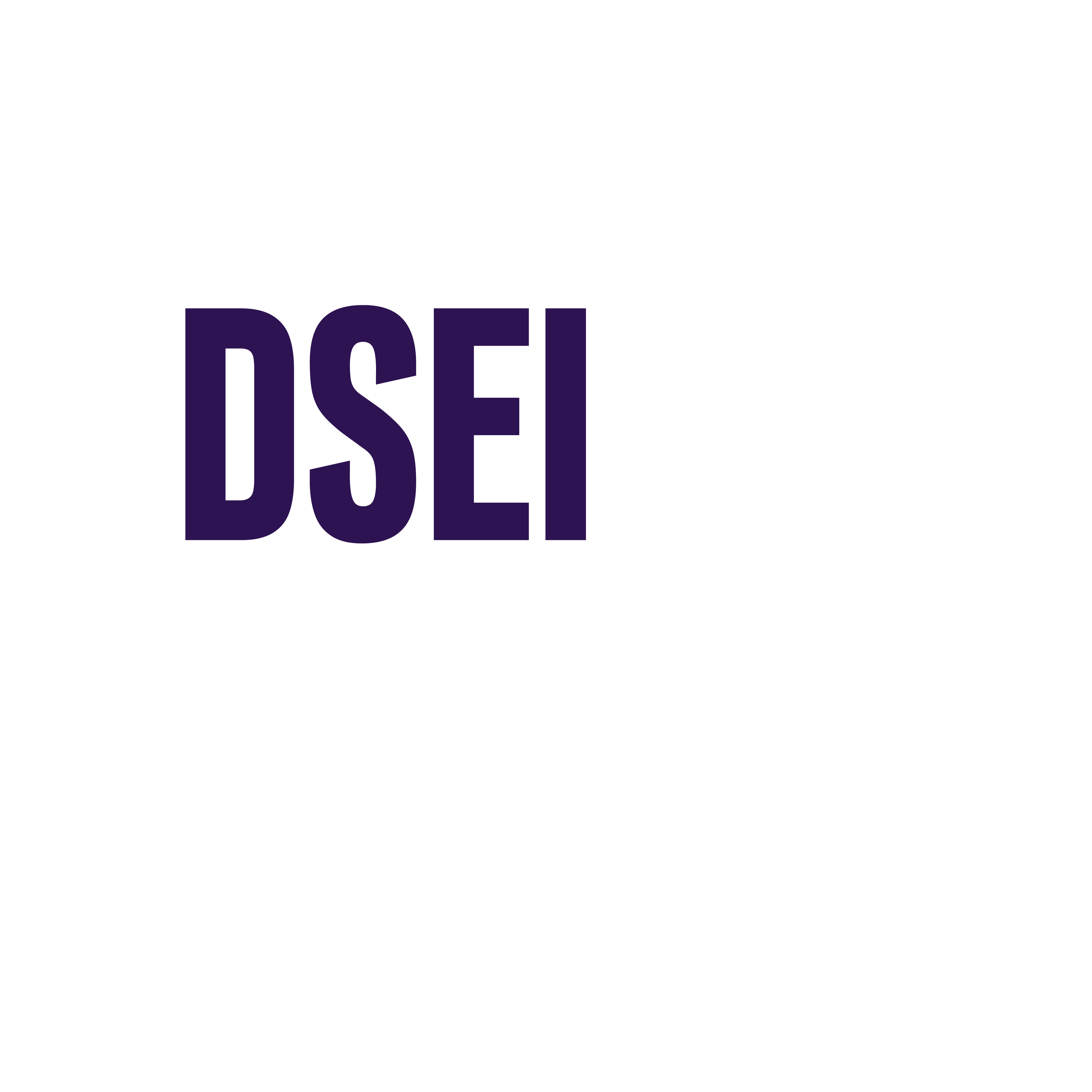
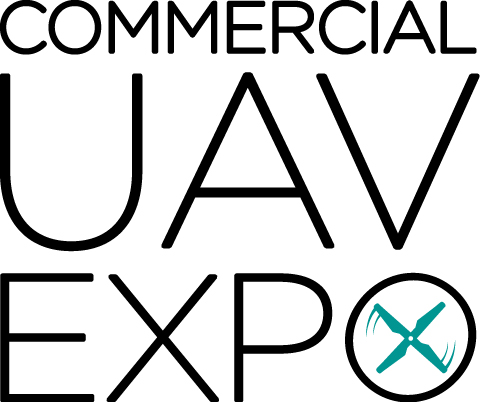
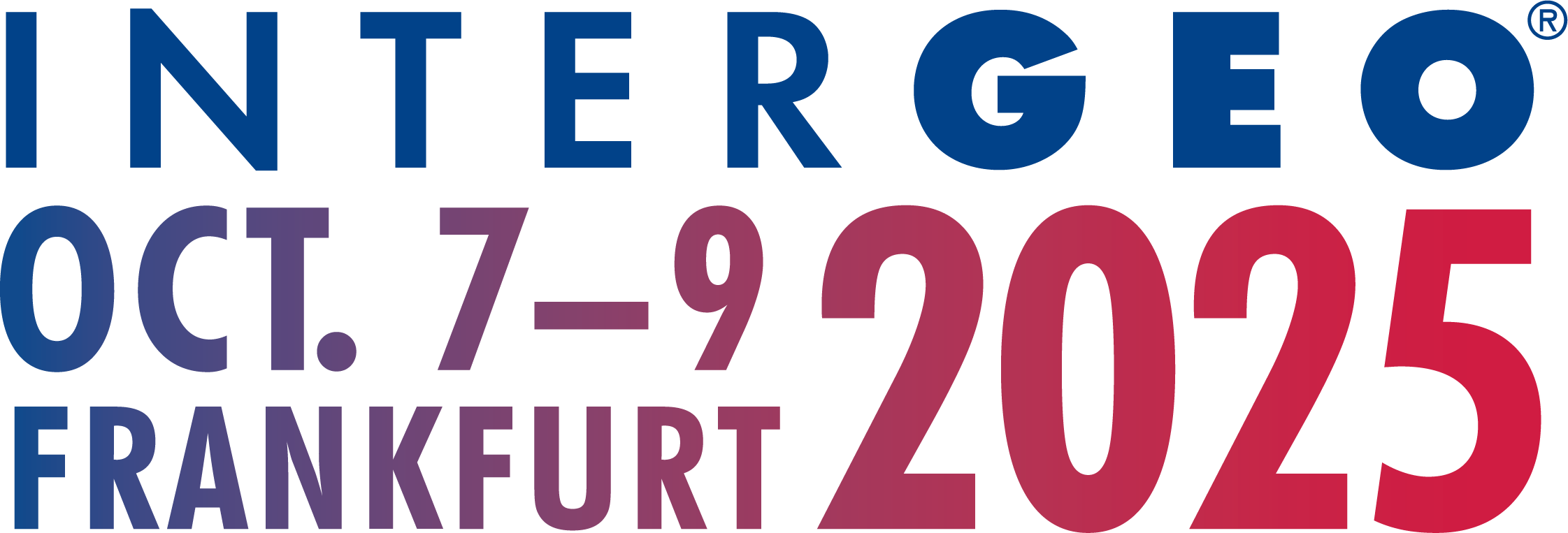
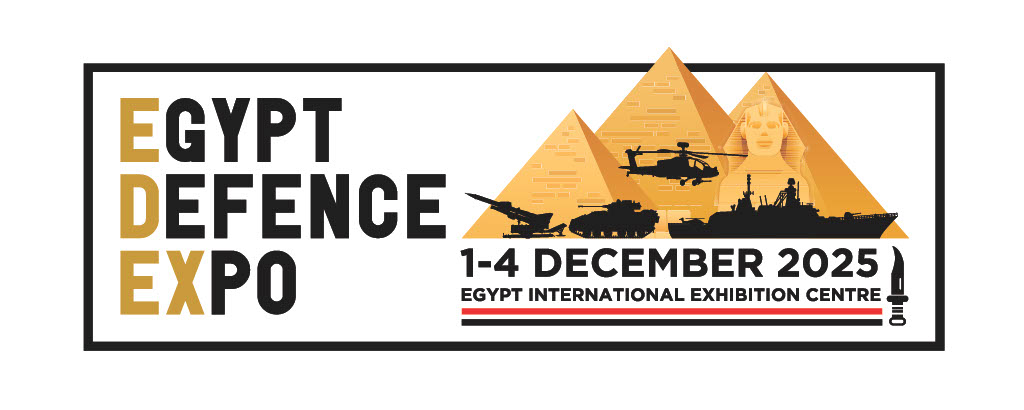
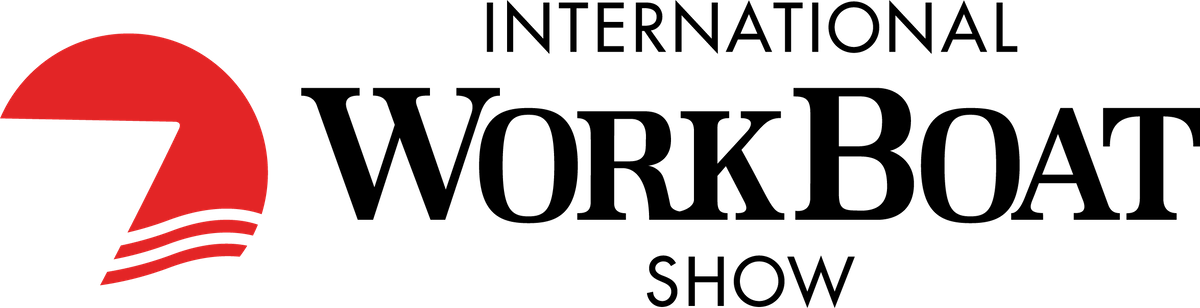